Self-absorption corrected non-invasive transmission Raman spectroscopy (of biological tissue)
Benjamin Gardner, Pavel Matousek & Nicholas StoneAbstract
The first near infrared window in biological tissue (λ ∼ 700–950 nm) is of great interest for its potential to safely deliver light based diagnosis and therapeutic interventions, especially in the burgeoning field of nano-theranostics. In this context, Raman spectroscopy is increasingly being used to provide rapid non-invasive chemical molecular analysis, including bulk tissue analysis by exploiting the near infrared window, with transmission Raman spectroscopy (TRS). The disadvantage of this approach, is that when probing depths of several centimetres self-attenuation artefacts are typically exhibited, whereby TRS spectra can suffer from relative changes in the “spectral features” due to differential absorption of Raman photons by the various constituents of biological tissues. Simply put, for a homogenous substance with increasing thickness, spectral variances occur due to the optical properties of the material and not through changes in the chemical environment. This can lead to misinterpretation of data, or features of interest become obscured due to the unwanted variance. Here we demonstrate a method to correct TRS data for this effect, which estimates the pathlengths derived from peak attenuation and uses expected optical properties to transform the data. In a validation experiment, the method reduced total Raman spectral intensity variances >5 fold, and improved specific peak ratio distortions 35×. This is an important development for TRS, Spatially Offset Raman Spectroscopy (SORS) and related techniques operating at depth in the near IR window; applicable to samples where there is large sample thickness and inter- and intra-sample thickness is variable i.e. clinical specimens from surgical procedures such as breast cancer. This solution is expected to yield lower detection limits and larger depths in future applications such as non-invasive breast cancer diagnosis in vivo.
"A Window to the Depths"
— by Ryan Edginton —
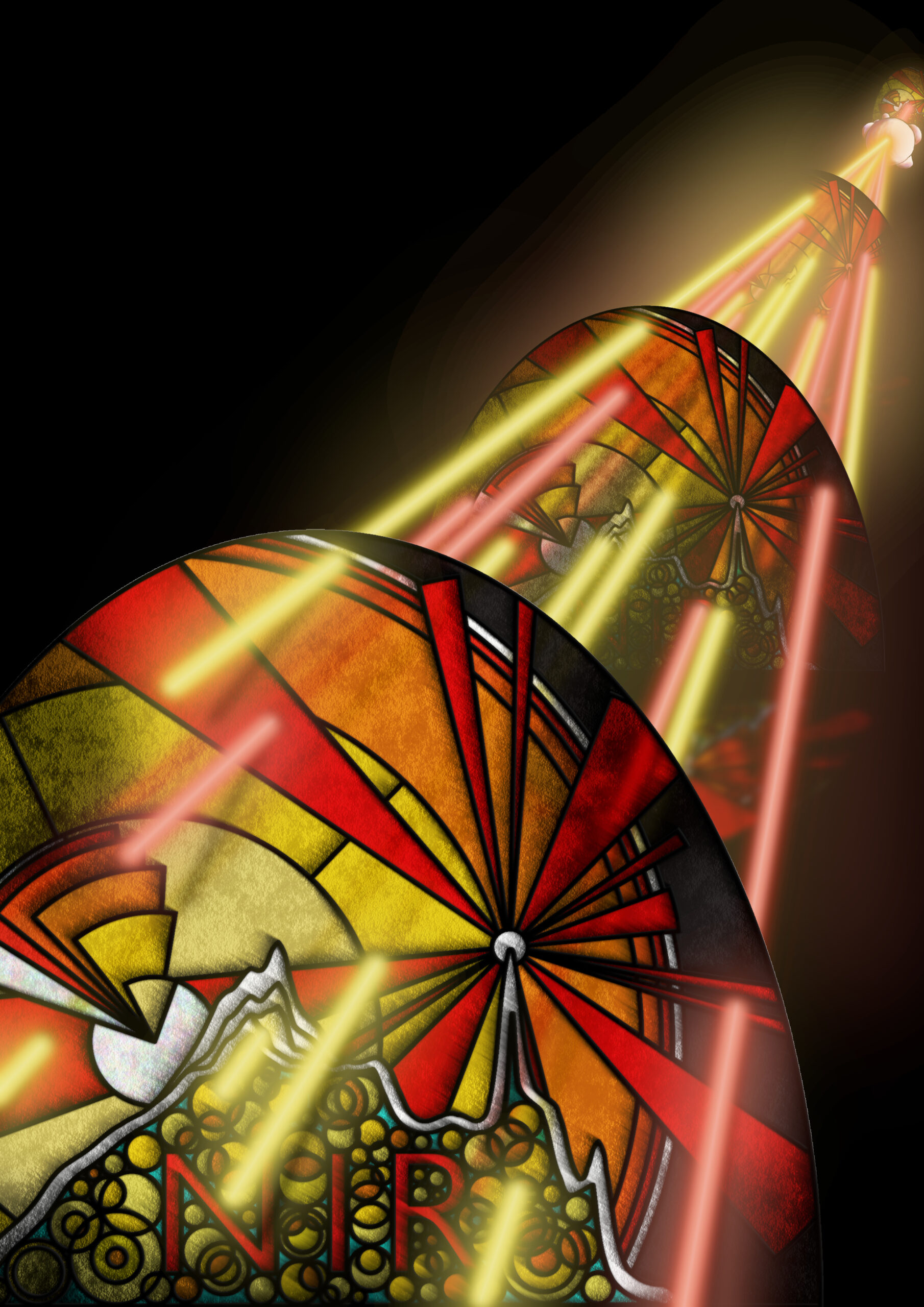
ABOUT THE IMAGE
Science Background
The near infra-red (NIR) optical windows are the wavelength ranges in which light penetrates deepest into the body. This is to say that our bodies are most transparent to red light a little beyond the visible spectrum. This is because the molecules that make up our tissues and life-fluids scatter and absorb less light at these wavelengths than for any other non-ionising radiation, so individual photons of NIR light can travel longer distances between molecules inside the body. Low powered NIR laser light can be used as a tool to see deep inside the body without piercing the skin or causing tissue damage.
A very small percentage of laser light experiences a slight, measurable wavelength change when scattered by a vibrating molecule; this is known as Raman scattering. Biological materials consist a collection of specific vibrating molecules that produce a distinct pattern of wavelength shifts unique to that biomaterial. This is like a chemical fingerprint and can be used to accurately identify the material.
A complication of measuring biological tissue deep inside the body in this way, is the myriad biomaterials contained in the intermediate space between internal target and external detector. These scatter and absorb the narrow spectrum of Raman scattered light by varied, wavelength-dependent amounts. The pattern of light wavelengths that reach the external detector, is therefore not the same as that measured directly at the target. This confuses the data and leads to possible misidentification of the internal biological tissue target.
Image Description
The artwork shows this wavelength-dependent variance in the transmission of Raman scattered light from-depth out of the body, resulting in non-chemical changes in the externally detected signal. The journal article describes a method developed to accurately correct for this effect.
In the background of the piece, a near infra-red laser beam is Raman scattered by a dense lump of cancer cells. 12 beams of light spread out from the tumour (6 red & 6 yellow; representing the spread of wavelength shifts contained in the scattered light), all travelling towards the foreground, passing through 3 stained-glass windows that differentially absorb the colour beams – 5 of the yellow beams reach you, the observer, but only 2 red beams successfully travel to this point.
The stained-glass windows are intended as a visual metaphor for the near infra-red optical window discussed above (hence the letters NIR on each pane). Choosing to layer the windows is representative of the depth and distance travelled by the Raman scattered light from the internal cancer to the surface of the skin, with increased absorbance potential at each stage.
The stained-glass contains depictions of light scattering (sunbeam pattern on the right), electronic and vibrational energy levels (concentric circles & enclosed triangles centred on the left), and the Raman spectra of lard, the sample studied in the journal article (undulating white line). The 880 cm-1 peak changes intensity whilst 1068 cm-1 remains fixed, reflecting the variable absorption of the Raman scattered wavelengths. Finally, overlapping golden orbs representing photons of light, fill the integral space beneath the spectra; the spectra being a count of the number of discrete Raman photons captured by the CCD camera detector.